Fertilizers
The KIS method relies on natural fertilizers created by the life in the system and the food we feed them. When setting up a system with the KIS method, it can take time for macro and microorganisms to reach an equilibrium state, producing the majority of nutrients consumed by plants. Some minor targeted supplementation may be required depending on the species of plants.
Based on scientific research, the optimal pH range appears to be 6.0 - 7.5 for nutrient availability for terrestrial plants. This range is for plants only; for determining an ideal range, the animals kept in the system must be considered. Many species of fish kept in the aquarium hobby will prefer a pH range higher or lower than the ideal range for plant nutrient availability.
The vast majority of scientific research done with nutrients and plants is with terrestrial plants; therefore, we can only use this information as an area to explore when attempting to diagnose nutrient deficiencies.
Microbes convert organic matter into bioavailable forms of key minerals, especially phosphorus, magnesium, iron, manganese, and sulfur. Detritus digesters (heterotrophic bacteria) make it possible to optimize the recycling of waste from fish as organic nutrients for plant growth. In the aquarium, microbes convert the phosphorus in fish waste into orthophosphates that aquatic plants can utilize.
The fish, plants, and nitrifying bacteria rely on the same recirculating water for optimum health and growth; hence water quality parameters must be favorable for all three organisms in the system.
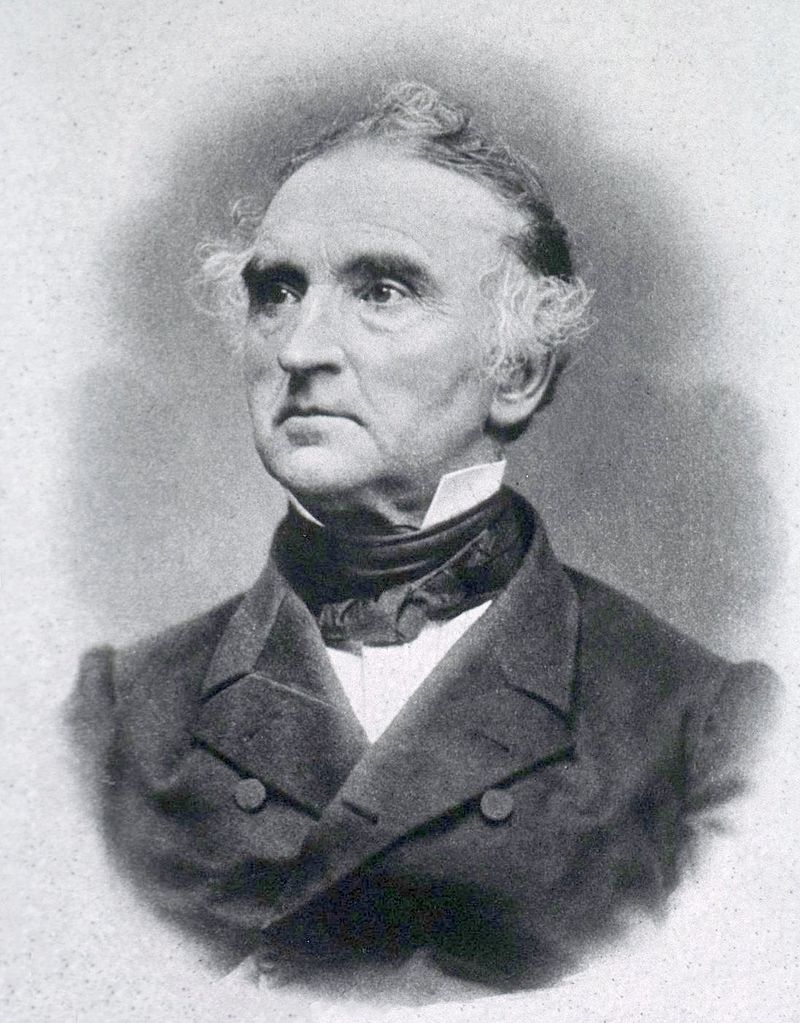
Liebig's law of the minimum is a principle developed in agricultural science by Carl Sprengel (1840) and later popularized by Justus von Liebig (12 May 1803 - 20 April 1873). Liebig was a German scientist and professor at the University of Giessen. Liebig devised the modern laboratory-oriented teaching method. Liebig made major contributions to agricultural and biological chemistry and is considered one of the principal founders of organic chemistry.
The law of the minimum describes how plant growth is limited by the scarcest nutrient resource rather than the total amount of resources available. The scarcest resource is the limiting factor in plant growth.
The law of the minimum takes into account all of the major and minor nutrients plants need. The law of the minimum was originally applied to plant or crop growth, where it was found that increasing the amount of plentiful nutrients does not increase growth. Only by increasing the amount of the limiting nutrient (the one most scarce in relation to "need") was the growth of a plant or crop improved. In aquatic environments, this also includes water chemistry.
All of the nitrogen (N), phosphate (P), and potassium (K) a healthy planted aquarium needs can come from source water (surface or groundwater), fish, and fish food added to the system. Nutrient deficiency issues are not very common in well-established systems (over a year old).
Nutrient deficiency should be targeted with specific nutrients to address the issue. The most common nutrient deficiency in systems that use the KIS method is iron (Fe). Some heavy root feeders, like Amazon swords (Echinodorus spp.), will benefit from Fe supplementation in the substrate. API's root tabs are an excellent substrate fertilizer for plants with a high Fe need.
Most of the science done on nutrients is with terrestrial plants. The terrestrial plant science can only be loosely applied to aquatic ecosystems. The nutrient needs of aquatic plant species vary. While hobbyists often focus on nutrient deficiencies, excess of a specific nutrient can also affect plant growth and health. Some nutrients in excess can inhibit the uptake of other essential nutrients.
Many hobbyists on the internet promote using Osmocote as a substrate fertilizer. Osmocote is formulated for terrestrial plants and therefore has excessive amounts of nitrogen and phosphate and is low in Fe. The excessive use of N in Osmocote increases methemoglobin in fish blood and could cause excessive algae growth because of the extra N and P.
For comparing fertilizers, manufacturers use the N-P-K ratio for major trace elements, which indicates the percentage of nitrogen, phosphorus (phosphate), and potassium. These numbers vary significantly between fertilizers. For example, the N-K-P ratio of API root tabs is 3-1-1 (3% N, 1% P2O5, 1% K2O).
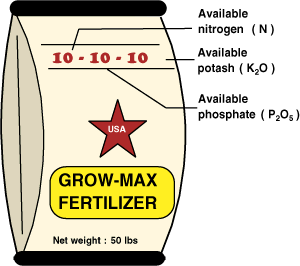
Fe is a minor trace element fertilizer, but for many aquarium plants, this nutrient is often targeted to bring out the color and is often a limiting nutrient for some species. API root tabs have 5% water-soluble Fe. Since planted aquarium hobbyists value iron, a N-P-K plus Fe (N-P-K+Fe) ratio should be considered when comparing aquarium plant fertilizers. For API root tabs, the ratio would look like this, 3-1-1+5. Fifty percent (50% by ratio) of the API root tab is iron, which could explain why it works so well with Echinodorus spp. For comparison, Osmocote Plus is 15-9-12+.46. Only .012% is Fe.
Excessive nitrate and phosphate levels can suppress reproduction, growth, and the fish's immune system. Nitrate and phosphate are pollutants monitored in natural waterways as they are directly tied to excessive algae growth.
"We concluded that both nitrates and phosphates have positive effects on algal growth. However, these variables affect algal growth independently of each other and there is no interaction between the two. This implies that both nitrates and phosphates are effective limiting nutrients that can be reduced to control algal proliferation[48]."
Nitrate and phosphate are naturally produced in aquariums and are typically available in excess. Avoid adding all-in-one fertilizers that contain nitrogen and phosphorus to the system when nitrate and phosphate test positive for adequate amounts. There are aquarium plant fertilizers that do not have nitrogen and phosphorus. Fertilizers that target iron and potassium are all the supplemental plant nutrition a KIS system may need.
The rate at which nutrients are used and the optimal level they should be present in a system is highly variable. Depending on water chemistry, pH, and plant species, the ideal levels for your system(s) can vary. Through experimentation and logging, a baseline can be developed. Systems change over time, and the number of nutrients added will change. There is no defined Goldilocks zone for all aquatic plants.
Research on aquatic plants and their nutritional needs is not an area of great scientific study. Most of the science is done on human food production crops. We can only extract some data from past research and loosely apply it to planted aquariums.
Research in aquaponics can help provide some insights into providing nutrients for aquatic planted aquariums. There are major differences between aquaponics and aquariums. The plants grown in an aquaponics system are typically terrestrial plants for human consumption. Plants grown in aquaponics systems can extract CO2 from the air. The water chemistry of a recirculating aquaponics system (RAS) is maintained to provide the maximum amount of nutrients for the crop while still being able to maintain fish health.
A decoupled aquaponic system (DAS) separates the fish from the plants. DAS adds old nutrient-rich water from the fish system to the hydroponics and adds targeted nutrient concentrations for the plants that would be toxic to fish.
Aquatic plants have evolved to extract nutrients from the water column and organic decomposition in the substrate. The availability of nutrients is often affected by pH and the ratios of other nutrients in the solution. Aquatic plants, like fish, have evolved in a specific range of GH (magnesium and calcium) and KH (bicarbonate and carbonate). Nutrients required for hydroponics plants may be higher than those adapted to fully submerged aquatic environments.
Aquatic plants require many essential macronutrients and micronutrients to grow. Most of these nutrients are provided by the fish feed, by-products produced by the fish, and decomposing plants. Humic substances (HS) that accumulate in aquatic systems have a beneficial role in aquatic ecosystems. HS is a source of organic nutrients and provides for the biological chelation of metals that plants can use. The chelation by bacteria in HS can also reduce metal toxicity to plants and fish.
Nutrients required for aquatic plant growth are listed below:
Macronutrients | Micronutrients |
---|---|
Nitrogen (N) | Chorine (Cl) |
Potassium (K)** | Iron (Fe)** |
Calcium (Ca) | Manganese (Mn) |
Magnesium (Mg) | Boron (B) |
Phosphorus (P) | Zinc (Zn) |
Sulfur (S) | Copper (Cu) |
Carbon (C) | Molybdenum (Mo) |
Hydrogen (H) | Nickel (Ni) |
Oxygen (O) | Sodium (Na) |
Silicon (Si) | |
Cobalt (Co) | |
Iodine (I) ^ |
The mobility of nutrients can play a role in plant health and appearance. Some nutrients can move from old parts of plants to new growth, while others are deposited and will not move.
Mobile nutrient deficiency symptoms appear first in older leaves; if the deficiency is not addressed, they can show up in new growth.
Immobile nutrients do not move to areas of new growth. Deficiency symptoms appear in the new growth first; if not addressed, they will appear in older leaves.
Mobile Nutrients | Immobile Nutrients |
---|---|
Nitrogen (N) | Calcium (Ca) |
Phosphorus (P) | Iron (Fe) |
Potassium (K) | Zinc (Zn) |
Magnesium (Mg) | Copper (Cu) |
Sulfur (S) | Manganese (Mn) |
Chorine (Cl) | Boron (B) |
Molybdenum (Mo) |
When tap water is used for a system, all the macro and micronutrients may be available in sufficient quantities for aquatic plants. The most common macro and micronutrients that may need supplementation are Fe and possibly K.
In most aquarium systems, nitrogen (ammonium, nitrate) and phosphorus (phosphate) are overabundant. Nitrogen and phosphate are typically reduced with water changes. If nitrate accumulates over time, the system is not balanced. Even if a nitrate test result shows 0 ppm, that does not mean there is no nitrogen; it only indicates that the nitrogen (created by fish expelling ammonia through their gills) is processed by NNR.
The uptake of ammonium can depress the uptake of potassium, calcium, and magnesium. When ammonium is the dominant form of nitrogen available for plant uptake, a smaller plant will result. Thus where the nitrogen source in the aquarium comes primarily from the fish, the nitrification process is essential for nitrate uptake by plants[50].
Freshwater aquarium test kits can measure nitrate, phosphate, potassium, and iron. At the time of writing this ebook, freshwater potassium test kits were not commonly available, and Salifert appears to be the only company that markets a freshwater kit.
Nitrogen
Nitrogen (N) is absorbed by plants to produce amino acids, proteins, enzymes, and chlorophyll. Nitrate (NO3) and ammonium (NH4+) are the most used nitrogen forms for plant fertilization. Nitrate is absorbed quickly by the roots, is highly movable inside the plants, and can be stored without toxic effects. Plants can absorb ammonium in low quantities but cannot be stored in high quantities because it exerts toxic effects. Ammonium above 10 ppm inhibits plant calcium and copper uptake, increases shoot growth compared to root growth, and results in a strong green color of the leaves[51].
Nitrogen deficiency is expressed as pale green color of the older leaves (chlorosis) and reduced growth. Fish are constantly producing nitrogen, so deficiency is rarely an issue in planted aquariums.
Phosphorus
Phosphorus/phosphate (P) stimulates root development, the rapid growth of buds, and flower quantity. Phosphorus is absorbed easily and can be accumulated without damage to the plant. Its fundamental role is linked to the formation of high-energy compounds (adenosine triphosphate) necessary for plant metabolism. The average quantities requested by plants are rather modest (10 to 15% of the needs of N and K).
The absorption of P appears to be reduced with increasing pH values (> 6.5), which can lead to deficiency symptoms. Excess phosphorus (> 10 ppm) can reduce or block the absorption of potassium, copper, and iron.
Phosphorus deficiency can show up as a green-violet color on the older leaves, which may cause chlorosis and necrosis in addition to stunted growth. These symptoms are non-specific and make phosphorus deficiencies difficult to be identified.
Potassium

Potassium (K) is required for cell division and extension, protein synthesis, enzyme activation, and photosynthesis and also acts as a transporter of other elements and carbohydrates through the cell membrane. It plays a vital role in keeping the osmotic potential of the cell in equilibrium and regulating the stomatal opening.
Potassium deficiency is manifested in the form of yellowish spots that quickly necrotize on the margins of the older leaves.
A water hyacinth (Eichhornia crassipes) study found the maximum nitrate and phosphorus storage in the plant biomass was reached at potassium (K) concentrations of 12 and 22 mg/l. Further increases in K concentration did not increase either N or P storage[52].
Potassium can be increased with potassium chloride (KCl).
Potassium Solution Formula
A potassium solution can be created with an empty water bottle:
Dose the system with 1 ml per 10 gallons (4 L) to raise the potassium level 1 ppm.
Calcium
Calcium (Ca) is involved in cell wall formation, membrane permeability, cell division, and extension. Calcium regulates nutrient transport and supports many enzyme functions. Good availability gives the plant greater resistance to disease. Calcium is not mobile within the plant.
Calcium deficiencies are not common in aquarium systems. If RO/DI water is used for water changes, ensure the replacement water is remineralized. Calcium deficiencies start from the most recently formed parts main symptoms are plant growth being stunted, deformation of the margins of the younger leaves, the light green or sometimes chlorotic coloring of new tissues, and a stunted root system without fine roots.
Magnesium
Magnesium (Mg) is used in the constitution of chlorophyll molecules. Magnesium contributes to the plant's ionic balance. At a pH below 5.5, magnesium is immobilized and competes with potassium and calcium absorption.
Magnesium deficiencies are not common in aquarium systems. If RO/DI water is used for water changes, ensure the replacement water is remineralized. Symptoms of magnesium deficiency are yellowing between leaf veins and internal chlorosis of the basal leaves. Magnesium can be quickly mobilized; magnesium-deficient plants will first break down chlorophyll in the older leaves and transport the magnesium to younger leaves.
Sulfur
Sulfur (S) is required by the plant in quantities comparable to those of phosphorus. To optimize sulfur absorption, it must be present in a 1:10 ratio with nitrogen (McCutchan et al. 2003). Sulfur is absorbed as sulfate. Magnesium sulfate (Epsom salt) is a readily available product that can target both magnesium and sulfate deficiencies.
Desulfosporosinus spp. anaerobic bacteria in the substrate produce hydrogen sulfide (H2S), which is then quickly converted to sulfate by Thiobacillus spp. bacteria. This process uses aerobic and anaerobic bacterial processes to naturally produce sulfate (SO42-) plants can use as a micronutrient.
Sulfur deficiencies are not easily detected, as the symptoms can be confused with those of nitrogen deficiency, except that nitrogen deficiency begins to manifest itself from the older leaves, while sulfur deficiency is visible in the youngest leaves. Sulfur nutrition has a significant role in repairing the damage in photosynthetic apparatus caused by iron deficiency.
Iron
Iron (Fe) is one of the most important micronutrients because it is key in many biological processes, including photosynthesis. Some studies of terrestrial plants suggest the optimal absorption of iron is achieved with a pH between 5.5 and 6.0. Aquaponics research shows a 6 to 8 is acceptable. The optimal Fe/Mn ratio is around 2:1 for most plants studied. A manganese (Mn) content should not be allowed to become too high because Fe and Mn enter into a competition.
In aquatic ecosystems, iron exists in two states, reduced ferrous ion (Fe II, Fe2+) and oxidized ferric ion (Fe III, Fe3+). In freshwater oxygen-rich systems, ferrous ion oxidizes to ferric ions. In freshwaters with a pH greater than 6.5, ferric ions are insoluble and vigorously precipitate as hydroxides and oxyhydroxides. To prevent the oxidation of Fe II, chelation is used to increase the time it is available in the solution.
Iron in a system can come from tap water and fish food.
Iron deficiency symptoms are expressed by interveinal chlorosis from the young leaves towards the older basal leaves and by reduced root system growth. Symptoms of iron deficiency are not always due to the low presence of the nutrient, but often they are due to the iron unavailability for the plant.
Some aquatic plant studies have shown iron distress at fairly low levels. 1.2 mg/l of Fe caused 75% reduction of growth in Potamogeton pectinatus (sago pondweed) and turned leaves brown[53]. Another study reported the best growth at 8 ppm of FeEDTA with Hydrilla verticillata[54]. This study also showed a decrease in manganese uptake. Hydrilla verticillata was reported as showing rusty brown color and decay at 1.2 mg/l[55].
The water chemistry in systems is unclear in studies about iron toxicity in aquatic plants. The ideal ratios of nutrients can be affected by pH, GH (calcium), KH, dissolved organic carbon (DOC), and metal concentrations.
Chelating agents like EDDHA and EDTA ensure constant iron availability for plants. EDDHA is stable between a pH of 4.0 to 9.0. EDTA is stable between a pH of 4 and 6[56]. EDDHA is a good iron chelate because of its effectiveness over a wide range of pH. EDDHA is stable in a recirculating aerobic system. In a study under hydroponic culture, Fe-EDDHA maintained more Fe in solution than Fe-EDTA for a period of 11 weeks.
Unfortunately, many of the iron fertilizers marketed by aquarium product manufacturers use Fe-EDTA. Fe-EDTA is not effective in the majority of the pH ranges hobbyists maintain in their systems.

Chelated iron is photoreactive (mostly light in the blue/UV spectrum) and temperature reactive (higher temperatures increase the degradation rate). As chelated iron degrades, it can increase the pH[57].
Adding chelated iron directly to the substrate while the system is dry or injecting an iron solution deep within the substrate can help protect it from light degradation. Using an ultraviolet sterilizer on a system dosed with chelated iron will destroy the chelation.
In planted aquariums, most bacteria and some plants respond to iron stress by introducing high-affinity Fe transport systems that utilize biosynthetic chelates called siderophores (sid-er-o-phores). To acquire iron competitively, some microbes have transport systems that enable them to use other siderophore types in addition to their own. Siderophores are produced in various microbes of the genera Pseudomonas, Bacillus, Enterobacter, Streptomyces, Gliocladium, and Trichoderma. Raaijmakers et al. (2010) reported that using Bacillus spp., Pseudomonas spp., and Streptomyces griseoviridis may prevent or diminish the effect of plant pathogens.
Pseudomonas spp. have an assortment of iron acquisition and regulatory genes, and they play a vital role in stimulating plant growth and controlling several plant diseases[58].
6% Fe-EDDHA Solution
Iron can be increased with 6% Fe-EDDHA. Hobbyists can create their own 6% Fe-EDDHA solution.
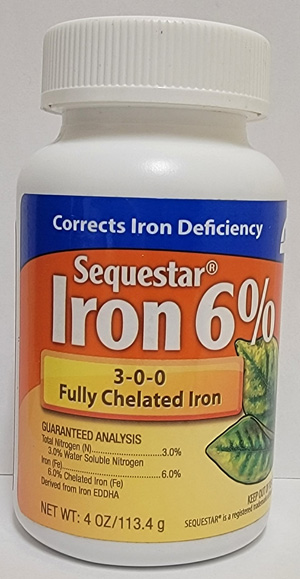
EDDHA Solution Formula
Have on hand a dark black or brown bottle that holds more than 500 ml. An empty hydrogen peroxide bottle can be reutilized for this purpose. Since chelated iron is photoreactive, it should only be stored in a dark-colored bottle.
Before adding a dose to the system, shake the bottle vigorously. Dose the system with 1 ml per 10 gallons (4 L) to raise the iron level .1 ppm. .2 ppm of iron is sufficient for most plants, but some may benefit from up to .5 ppm. Sera recommends not exceed .5 ppm. Use a syringe to inject the solution deep into the substrate.
When using 6% Fe-EDDHA solution to increase iron in the system, be aware that it will add a pink color to the water column.
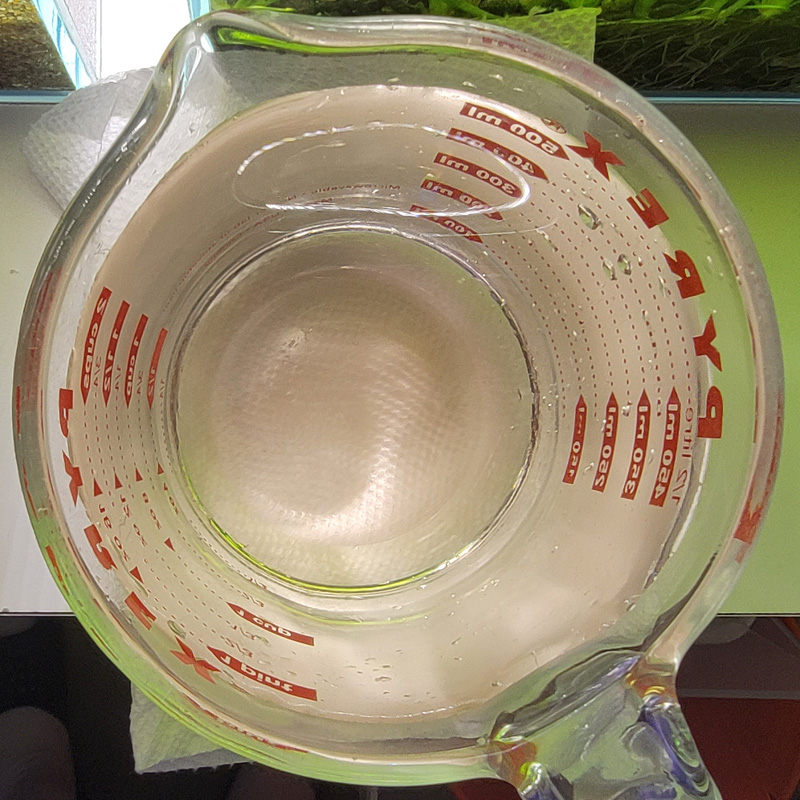
Fe-EDDHA is commonly available in dry form at local garden centers (nurseries).
Excessive iron in the water column may stimulate undesirable algae growth. Inject the iron solution deep within the substrate to prevent excessive iron in the water column.
Due to the risk of causing Fe metal toxicity and excessive algae growth, iron should only be dosed in tiny amounts in the substrate to target plant iron deficiency symptoms.
Nitrogen (nitrate) and phosphorus (phosphate) can be considered the gasoline for algae growth, and iron is the flame that starts the combustion.
In a system maintained for years with Cryptocoryne walkeri “Lutea” and Sagittaria subulata that had zero algae issues, when the excess C. walkeri were removed for a local fish club auction, some old iron-rich root tabs were released to the water column. Within a week, staghorn algae grew profusely on the tops of the remaining C. walkeri. After several near 100% water changes over the course of several months, the algae disappeared.
It can take up to two months before hobbyists notice increased plant growth and health after adding additional Iron.
Carbon
Carbon (C) can make up to 50 percent of the dry weight of plants. All plants get carbon from carbon dioxide (CO2), and some can get it from bicarbonate (HCO3) in the aquarium (Vallisneria spp.).
CO2 deficiencies are not common, but having strong surface agitation can help with gas exchange and add CO2 to the solution. Strong surface agitation can also drive excess CO2 from the system that is being produced by bacteria in the substrate.
Systems with strong surface agitation and systems with minimal surface agitation can support good plant growth. When choosing plants to maintain in a system, hobbyists should consider the natural ecosystem where they are found. This will ensure the plants are adapted to the CO2 and oxygen concentration in the aquarium. As with other gases, the CO2-carrying capacity of water is affected by temperature, altitude, and chemistry. Warmer water holds less CO2. Higher altitudes and harder water also reduce the CO2 capacity. Most freshwater can hold 3 to 4 ppm CO2[59].
Dissolved organic carbon (DOC) is a compound that is the result of decomposition processes from dead organic matter, including plants and animals. In the aquarium, DOC can be decaying plants and algae, wood, leaf litter, and accumulated detritus. DOC is a basic nutrient supporting growth of microorganisms and plays an important role in the carbon cycle through the microbial loop. Dissolved organic carbon has a high proportion of biodegradable dissolved organic carbon (BDOC). Heterotrophic bacteria use BDOC as a source of energy and carbon. The decomposition of organic carbon releases inorganic carbon (CO2) into the aquarium ecosystem that can be either used by plants or escape into the atmosphere.
Organic carbon can be divided into particulate organic carbon (particles > 0.45 μm) and dissolved organic carbon (particles < 0.45 μm). In nature, DOC usually makes up 90% of the total aquatic organic carbon.
Chlorine
Do not confuse the chlorine element (Cl) with the more complex household bleach (sodium hypochlorite [NaOCl]) or the disinfectant added to municipal water supplies. Chlorine/chloride is considered a micronutrient even though the dry weight content in plants is .2 to 2%. It is easily absorbed and is mobile within the plant. It is involved in the photosynthetic process and the regulation of the stomata opening.
Deficiencies of chloride are rare and with typical symptoms for emersed plant leaves drying out, especially at the margins. Also rare is damage from excess chloride, which causes conspicuous plant shrinkage relative to the different sensitivities of different plant species. If excessive chloride content in the water is suspected, use potassium sulfate (K2SO4) instead of potassium chloride (KCl) for a nutrient solution. The maximum chloride in the solution for a hydroponics system is reported to be 531 ppm, which is unlikely to be reached in freshwater aquariums with regular water changes.
Sodium
Sodium (Na), if in excess, is toxic to plants. Excessive sodium interferes with the absorption of other ions. It is in opposition to potassium.
Sodium is beneficial at low levels, but at high concentrations disturbs and inhibits various physiological processes and plant growth. The chemical similarities between potassium (K) and Na allow some of the functions of potassium to be undertaken by sodium. High concentrations of cytosolic sodium (fluid in cells) are toxic to plants. The major role of Na is considered to be the regulation of turgor pressure (hydrostatic pressure) and cell expansion[60].
Manganese
Manganese (Mn) forms part of many coenzymes and is involved in root cell extension and disease resistance. The availability of manganese is affected by the pH and competition with other nutrients. Manganese becomes more bioavailable at a lower pH (4.5 to 6.5), but it also will be in competition with other nutrients with a lower pH.
Manganese deficiencies are similar to those of iron except for the appearance of slightly recessed areas in the interveinal areas. Adding manganese sulfate (MnSO4) or lowering the pH can help solve deficiencies. Adding additional calcium to the water may prevent manganese toxicity[61].
Boron
Boron (B) is essential for fruit setting and seed development. The boron absorption competes with calcium. Boron is involved in plant carbohydrate transport and assists in metabolic regulation. The role of boron in aquatic plants or aquaponics is an area that needs more study. Some aquaponics studies show negative effects of boron at a pH of 7.2 and weekly added at 0.01 mg/L[62].
Terrestrial studies suggest the pH must be below 6.0, and the optimal level is between 4.5 and 5.5. These low pH levels are not common in planted aquariums or aquaponics.
Boron deficiency symptoms can be detected in the new structures that appear dark green. The young leaves greatly increase their thickness and can have a leathery consistency. Leaves can appear chlorotic and then necrotic, with rusty coloring.
Zinc
Zinc (Zn) plays an important role in specific enzymatic reactions. Its absorption is influenced by the pH and the phosphorus (phosphate). pH values between 5.5 and 6.5 promote the absorption of Zn. Low temperatures and high phosphorus levels reduce the amount of zinc absorbed by plants.
Zinc deficiencies are rare and are represented by chlorotic spots in the interveinal areas of the leaves, very short internodes, leaf epinasty, and poor growth.
Copper
Copper (Cu) is involved in respiratory and photosynthetic processes. The absorption of copper is reduced at pH values higher than 6.5, and pH values lower than 5.5 may result in toxic effects. High levels of ammonium (NH4+) and phosphorus (P) interact with copper reducing the availability. The excessive presence of copper interferes with iron, manganese, and molybdenum absorption.
With emersed plants, copper deficiencies are expressed by interveinal chlorosis, which leads to the collapse of the leaf tissues that look dried out.
Molybdenum
Molybdenum (Mo) is essential in protein synthesis and nitrogen metabolism. The availability of molybdenum is ideal at 7.0 pH.
Symptoms of molybdenum deficiency start with chlorosis and necrosis along the main rib of old leaves, and young leaves will appear deformed.
New setup systems often have low nutrients and microbial activity and can benefit from initial substrate augmentation with a dry fertilizer (root tabs). It can take months to more than a year for a new aquarium to reach an equilibrium state, where all the microbiology and macroorganisms produce most, if not all, nutrients aquatic plants need to thrive.
Plants will often go through an initial shock of being added to a new system, and it can take up to two months before they completely recover.
One technique to help bridge the gap of available nutrients from a new to a biologically mature system is to grind up root tabs in a mortar so they can be added to the substrate.
While the system is dry, add 50% of the sand (1 inch or 2.5 cm) to the bottom of the display. Add powdered root tabs to the sand and mix in thoroughly and flatten the sand. This technique will help keep most of the nutrients in the substrate. Add the rest of the clean sand on top.
It is recommended that hobbyists only add half the recommended amount of root tabs for a given area to minimize the chance of over-fertilization and excessive algae.
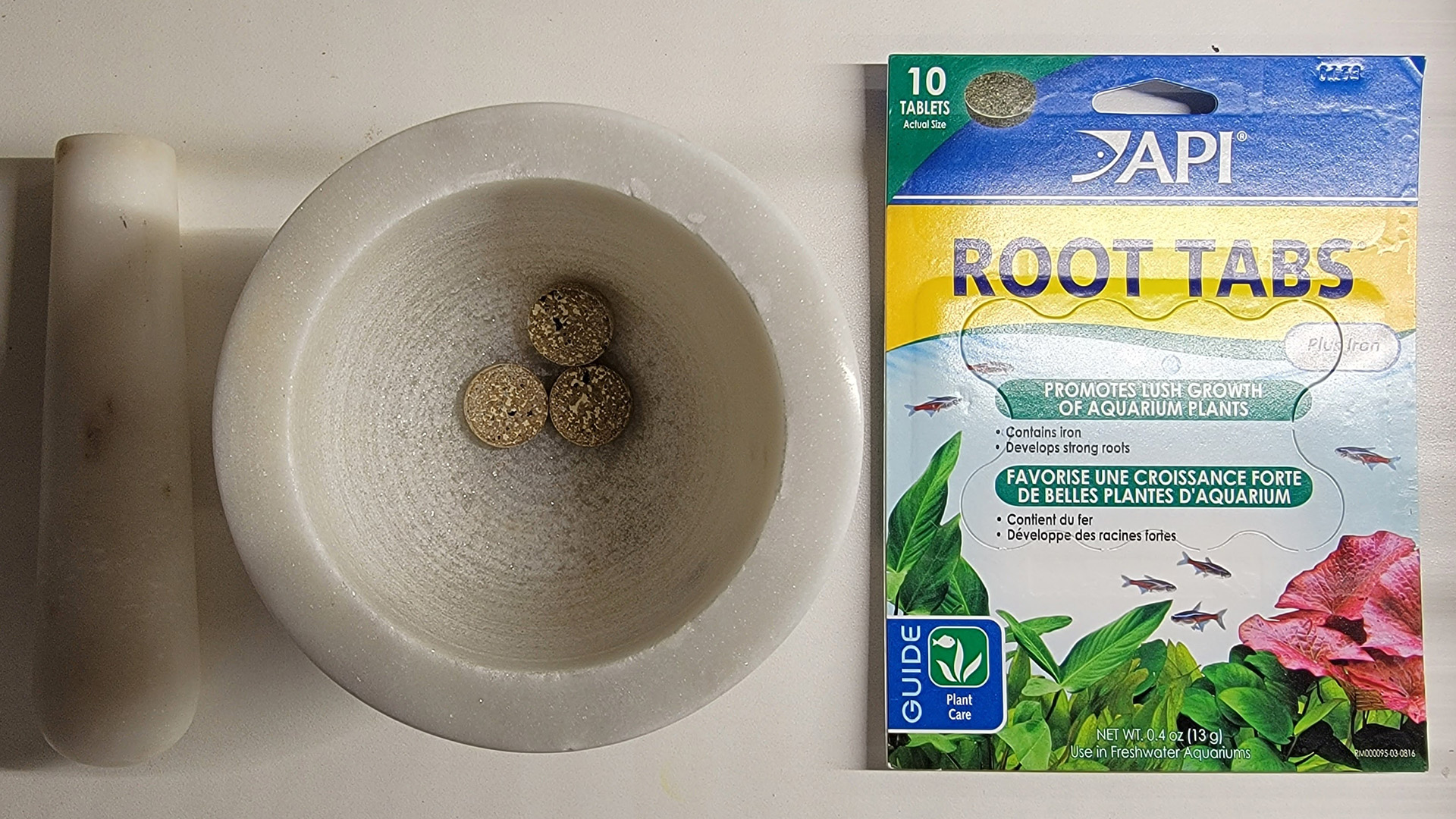




